Day 2 :
- Sequencing | Structural Molecular Biology | Structural Biology in Cancer Research| Signalling Biology
Location: Alto
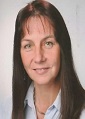
Chair
Annette G Beck-Sickinger
Leipzig University
Conference Series Ltd invites all the participants across the globe to attend 10th International Congress on Structural Biology Helsinki, Finland.
Leipzig University